Visible
Light Quality - its spectrum and its colour.
Since the development
of the first electric lamps 130 years ago, the market for lamps for
human and animal lighting has become confusing. To make the choice for
customers easier, the correlated colour temperature (CCT) and the colour
rendering index (CRI) of the lamp are often provided. But what meaning
do these numbers have for reptile keepers?
The correlated
color temperature (CCT) is an indicator of the colour the light appears
to be (to humans); the colour rendering index (CRI) of a light source
is an indicator of how "true" the colour of objects illuminated
by the light will appear to the human eye.
Correlated
Colour Temperature (CCT)
As the temperature
of an object increases, it radiates electromagnetic energy which at
first is invisible - infrared radiation - but which, at a certain temperature,
includes visible light.
In other words, it is so hot that it begins to glow.
As the temperature rises yet further, shorter wavelengths are included
in the emission, and so the colour of the light changes from deep red
through orange, yellow, white, and finally blue- white at very high
temperatures. Even hotter, and UVA is emitted... then UVB, and so on.
Even UVC can be recorded from the glowing tungsten filament in an unshielded
halogen lamp.
Physicists have
invented the concept of a black body - an idealised object that absorbs
all radiation falling on it, at all wavelengths. This is also known
as a Planckian radiator. When a black body is at any specified temperature,
its radiation - called blackbody radiation - has a characteristic, known
spectrum and, if the temperature is high enough, a very specific colour.

The chart above shows the
colours of blackbody radiation and their associated temperatures in
degrees Kelvin (K). The correlated colour temperature (CCT) of a light
source is the temperature of an ideal black-body radiator emitting light
that appears to be closest to the colour of that light source - as
seen by a human observer. Lower colour temperatures (up to about
3,000 K) are called "warm colours" (the light has a red or yellow hue)
whereas higher colour temperatures (above about 5,000 K) are said to
be "cool". This confuses many people, but it is because we associate
red with fireside warmth, whereas blue brings snow and ice to mind,
not blue-white heat.
The sun is not a black body,
but daylight is considered to have a spectrum similar to that of a black
body with a CCT of 6,500 K.
Colour Rendering
Index (CRI)
The colour rendering
index (CRI) is an assessment of the ability of the light from a lamp
to render (i.e., to reproduce) the colours of whatever it illuminates
faithfully to the human eye, as compared to a reference light
source.
The International Commission on Illumination defines colour rendering
as: "The effect of an illuminant on the color appearance of objects
by conscious or subconscious comparison with their color appearance
under a reference illuminant."
For lamps with a low colour temperature (below 5,000K) the reference
light source is a Planckian radiator. For lamps with a higher colour
temperature, which are intended to mimic daylight, the "natural light
source" with which the light is compared is one of a series of CIE 'standard
illuminant D' (daylight) reference spectra chosen to match the lamp's
CCT as closely as possible. 8 different test colour samples chosen to
represent a wide range of hues (R1 - R8 - see the chart below) are illuminated
by the lamp and scored according to the degree of difference between
the resulting apparent colour, and that of the sample when lit by the
reference lamp. A high score, close to 100, indicates a good match.
The average (mean)
of all 8 scores gives the general CRI (Ra) - usually just shortened
to "CRI".
Some lamps -
especially those used in situations where saturated colours and flesh
tones must be rendered well, such as in portrait or colour design work
- are further tested with another six colours (R9 - R14). Bright red
(R9) is a colour which is particularly difficult for fluorescent lamps
and mercury vapour lamps to render well.
The highest
CRI scores, not surprisingly, are achieved with light sources with continuous,
or nearly continuous spectra that resemble sunlight, such as metal halides.
However, if discontinuous spectra have peaks in the right wavelengths
to stimulate the tri-chromatic human visual system in the optimal way,
a very high CRI can be achieved. This is why some "tri-phosphor" fluorescent
tubes have a CRI of 90 or more. However, reptilian visual systems
are very different. It is unlikely that these spectra produce "white
light" to reptile eyes. This will be discussed in detail below;
see also our feature on reptile vision.
CRI
and CCT – meaningful numbers for herpetology?
The
article that follows was published in German as: WUNDERLICH, S. (2012):
Farbtemperatur und Farbwiedergabe – Sinnvolle Größen in der Tarraristik.
– Terraria/Elaphe 37: 40–47.
Lamps with a
colour temperature of 3,000 K are classified as ideal for the living
room, because they seem comfortably warm to us. For the office, colour
temperatures between 4,000 K and 6,000 K are recommended, because they
are closer to natural daylight and believed to support attention and
concentration. Under certain lamps colours of objects seem unnatural
and wrong. Whenever good colour rendering is needed, for example for
artistic work or when selling clothes or food, lamps with a high colour
rendering index are recommended.
In reptile keeping,
these recommendations are often applied intuitively. Often one can read
that a good terrarium lamp should have a colour temperature between
4,000 K and 6,000 K and a colour rendering index as high as possible.
But reptiles have very different colour vision from humans. Are CCT
and CRI therefore really meaningful, when choosing a terrarium lamp?
The Mathematics
of Colour
The
CRI and CCT aim to measure the colour properties of a lamp. To understand
them it is necessary to take a look at the concept of “colour” first.
The human eye contains three types of cones that react to blue, green
and yellow light with an electric signal. They are also called 'blue',
'green' and 'red' cones. (Figure 1). When white light shines
into our eye, the three cones are stimulated with the same strength.
The retina sends the signal “white” or “bright” to the brain. Only when
the three cones are stimulated with different strength, “colour” is
sent as a signal to the brain.
The colour impression
does not only depend on the relative excitation of the three cones,
but also on the absolute intensity and the colour stimuli from the surrounding.
This is, however, neglected in the calculation of CCT and CRI.
A typical white LED stimulates
the three cones with the ratio blue : green : red = 0.39 : 0.33 : 0.28
(Figure 2). This ratio is written so that the sum is one, i.e: 0.39
: 0.33 : 0.28 = 1. This is always possible to calculate. (For example,
the ratios 4 : 16 and 0.2 : 0.8 are identical.) Subsequently one can
omit one of the numbers, and the other two can be used as an x-y-coordinate.
The ratio of the cones' excitation 0.39 : 0.33 : 0.28 or the coordinate
(0.28;0.33) determine what colour we see. The eye cannot distinguish
between two lights with different spectra that stimulate the cones in
the same ratio. The colour is perceived as identical. To compare colours,
the colour coordinates are drawn in a chromaticity diagram known as
the CIE-colour-triangle (Fig. 2B). All colour calculations are based
on this representation of colour as a point in an x-y-diagram.
In this diagram
are also plotted the colour coordinates of light that consists of a
single wavelength. They form the spectral locus - the sides of the triangle.
They act as reference when comparing colours. For example, we see colour
coordinates that lie close to the colour coordinate of 650 nm as red,
and colour coordinates close to 550 nm, as green. A second reference
point is the white point that corresponds to a spectrum with constant
intensity. It lies at (1/3;1/3).
The LED's co-ordinates (in this example) are shown as a black square
in the CIE colour triangle. It is close to the white point, but shifted
a bit towards the turquoise part of the spectral locus. The colour of
the LED is white, with a very slight turquoise tint.
The colour diagram
is not based on the sensitivity of the three cones, but on color matching
functions, which are a linear transformation of the cone sensitivities.
In the case of the LED (Figure 2), however, the values hardly deviate
from each other.
Often
the colour coordinates are given in the manual of a lamp, but are too
complex for the customer. The so-called Correlated Colour Temperature
(CCT) is easier to understand. As described above, it results from
a comparison with the colour of a Planckian radiator ('black body radiator').
Figure 3 shows the colour coordinates of Planckian radiators with temperatures
between 1,000 K and 40,000 K. They form an arc across the CIE-colour-triangle
(shown here in black).
To calculate
the colour temperature of a lamp, one first draws the colour coordinate
of the lamp into the colour diagram and then searches for the nearest
point on the Planckian locus. The colour coordinate of the LED shown
here lies close to the colour coordinate of a Planckian radiator with
6,500 K, therefore the LED is said to have a correlated colour temperature
of 6,500 K. Nevertheless, the two light sources do not have the same
colour, and the two spectra can be totally different; it is only to
the human eye that the colour impression is very similar.
The calculation
of the Colour Rendering Index (CRI) of a lamp is a bit more complex.
In the first step, a second lamp with the same Correlated Colour Temperature
is needed as a reference. This is either a Planckian radiator or a theoretical
model of daylight. In the second step, the 14 test colour samples (described
above) are irradiated with the lamp and the reference lamp and the colour
coordinates of the reflected light marked in the colour diagram. If
all colour samples have the same colour coordinate when irradiated with
both the lamp and the reference lamp, the lamp has a colour rendering
index of Ra=100.
Colour
Psychology and Natural Daylight
Scattering and
filtering of light in the atmosphere, in clouds, or reflected from the
environment (foliage, sand, rocks) influences the colour of daylight.
The spectra of some of these different forms of natural light are shown
in figure 4. To the human observer, the scattered light from the sky
that reaches the ground in the shade of a rock or trees is bluish. Under
dense foliage, neither direct sunlight nor skylight reach the ground,
but only the greenish light that is filtered by the leaves (ENDLER 1993;
FEDERER 1966; DECASTRO 2000). At sunrise and sunset the light is reddish
and “comfortably warm”, whereas bright sunlight is "warm-white"
with 5,000 K colour temperature. When it is cloudy, the light becomes
"cool-white", around 6,500 K. In foggy weather, or in daylight
with snow, the colour temperature may reach “cold and uncomfortable”
values of up to 20,000 K.
There is a palpable
connection between our perception of low colour temperatures as 'warm'
and high colour temperatures as being 'cold', and the actual temperature
in the respective situations under natural daylight, as illustrated
in Figure 5. Reptiles, as poikilothermic animals, depend much more on
the weather than man does. Could this mean that they are also more sensitive
to the different coloured phases of daylight?
Colour vision
in reptiles
There are two
ways of studying whether reptiles are able to see colours, that is,
whether they are able to distinguish differently coloured lights independent
of their intensity.
Firstly, one can train a reptile to distinguish between two differently
coloured plates. In subsequent tests, the colours of the two plates
are made increasingly similar, until the reptile is no longer successful
in choosing the right plate. This demonstrates how well it can distinguish
two colours.
The second method is to study the retina: What cones are present in
the retina, and what wavelengths of light are necessary to make them
respond with a nerve signal?
Colour
vision of reptiles has been studied in turtles (AMMERMÜLLER & KOLB 1996;
ARNOLD & NEUMEYER 1987), chameleons (BOWMAKER & LOEW 2005), geckos (TAKENAKA
& YOKOYAMA 2007; ROTH & KELBER 2004), anoles (LOEW et al. 2002), snakes
(SILLMAN et al. 1997) and salamanders (PRZYREMBEL et al. 1995). In general
it can be said that many reptiles have four cones, that are sensitive
to UVA, blue, green and red. Some species, especially night active and
crepuscular species, lack the red cone (TAKENAKA & YOKOYAMA 2007; SILLMAN
et al. 1997). The four cones in a hypothetical “archetypal” reptile
eye (devised for this illustration) are shown in Figure 6. Four cones
enable tetrachromatic colour vision, in contrast to the trichromatic
colour vision which results from the three cones in the human eye.
Terrarium
lighting
In the terrarium,
fluorescent tubes, metal halide lamps (“hqi”), mercury vapour lamps
(“hql”), mixed light lamps (also known as internally ballasted mercury
vapour lamps), incandescent lamps and halogen incandescent lamps, as
well as the new LEDs are used.
Figure
7 shows the spectra of three typical fluorescent tubes - one UVB-emitting
tube designed for the terrarium (Namiba Terra UV Replux); one "full
spectrum" lamp for human use which emits traces of UVA and UVB
(Narva BIOvital) and one "daylight" tube for human use (Osram
Lumilux 840). Fluorescent lamps are available in the form of long tubes
as well as compact lamps. The mercury vapour in a fluorescent lamp emits
mainly UVC and small amounts of UVA (365 nm), blue (405 nm and 436 nm),
green (546 nm) and yellow (578 nm) light. The main part of the light
comes from the fluorescent phosphors that convert UVC radiation into
visible light or UVA and UVB. Remaining UVC is absorbed in the glass.
The usual tri-phosphor lamps with colour rendering index >80 contain
three different phosphors. Their relative amounts can be adjusted to
set the colour temperature. The spectrum is quite discontinuous, consisting
of the mercury lines and the slightly wider lines of the phosphors.
Newer “de-luxe” phosphors offer a more balanced and sometimes very continuous
spectrum. The CRI of these lamps is often higher than 95. Sometimes
these lamps also emit the UVA that is visible to reptiles.
Also in figure
7 is the very discontinuous spectrum of a typical non-UVB mercury vapour
lamp sold for human use. These (“hql”) are operated with a higher pressure
than fluorescent tubes, so that the visible mercury lines become brighter.
Sometimes one phosphor is added, to cover the red spectral range. UVB-emitting
mercury vapour lamps are still widely used in the terrarium, often combined
with a incandescent filament, which also acts as a ballast.
Mercury vapour
lamps are often classified as out-dated and are now widely replaced
by metal halide (hqi) lamps (Figure 8, below), that have a more continuous
spectrum and a higher efficiency. The lamps illustrated are two UV-emitting
examples, the Lucky Reptile Bright Sun UV Jungle (BS Jungle); the Lucky
Reptile Bright Sun UV Desert (BS Desert); and two modern halides designed
for human use - a ceramic halide (HCI) and a quartz halide (HQI).
Early metal
halide lamps, whose spectrum was still quite discontinuous, contained
the metals sodium, thallium and indium additionally to mercury. Today
the UV-emitting metal halide lamps sold for terraria are still of this
type, with quite discontinuous spectra and a higher proportion of UVA.
However, the filling of modern quartz or ceramic lamps with a complex
blend of rare earths may result in a very solar-like spectrum.
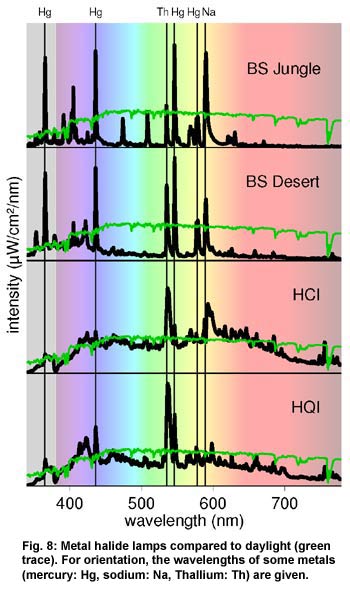 |
|
Figure 9 (above)
shows the spectra of a traditional incandescent lamp ("white light
bulb") and two white LEDs. LEDs
are only sporadically used in terraria at present. White
LEDs are usually semiconductor diodes that emit blue light, where a
part of the blue light is converted by a phosphor (as in fluorescent
lamps) to yellow light, so that the light seems white to the human eye.
These lamps promise a very high efficiency, especially compared to incandescent
lamps and compact fluorescent lamps. However, fluorescent tubes and
metal halide lamps are still more efficient in most cases, and above
all, they are favourably priced.
Incandescent
lamps and halogen lamps emit an absolutely continuous spectrum, which
is, however, mainly concentrated in the infrared-A and contains little
visible and very yellowish light. They are mainly used as a heat source
for the basking area.
CCT and CRI
recommendations for the terrarium
Lamps are usually
chosen by their colour temperature and their colour rendering index.
Many reptile keepers follow recommendations from the internet or found
in the literature. Here a high CRI is often correlated with a natural
light spectrum, or a CCT of 6,000 K is demanded. But is this really
reasonable?
Regarding CRI,
one website states “The Ra-Number indicates how natural the spectrum
of the lamp is. A high number, for example 8xx (= 80 Ra) or 9xx (= 90
Ra) signifies that the composition of the light is very close to sunlight.”
Horn (2004) writes “The choice of suitable lighting for the husbandry
of reptiles … should always be geared to the irradiative properties
of the sun as a basis for comparison. In conformity with these demands,
the manufacturers of artificial lamps point to products with a continuous
emission spectrum and a colour temperature of 6,000 K.”
Even if this assumption is not formulated in such striking terms, the
volume of text that is dedicated to CCT and CRI in husbandry guides
alone (ca. 10% in KOBER & GEISSEL 2006) suggests that these numbers
are essential for reptile keeping.
The aim of such
recommendations is to find a lamp whose light is as close to natural
daylight as possible. As long as we do not know whether reptiles react
in their behaviour or wellbeing to the colour properties of light, this
aim is certainly not wrong. Also, nearly all reptile keepers are aware
of anecdotal observations that a reptile will prefer any natural sunlight
falling into the terrarium to the expensive lamp inside!
Many species seem much more active, even as far as having better reproductive
success, if they are kept in natural daylight, whether this is outdoor
residence in the summer or simply a terrarium close to a window (window
glass is transparent for the UVA that is visible to reptiles). UVA content
in the illumination serves intraspecific communication and also plays
a role in mating (ALBERTS 1989; FLEISHMAN et al. 1993; LEAL & FLEISHMAN
2002; LEBAS & MARSHALL 2000).
However, the
colour vision of reptiles is fundamentally different from human colour
vision. The calculation of CCT and CRI always depends on the spectral
sensitivities of the three cones in our human eye. Therefore it is very
unlikely that a method that is so linked to human colour vision should
give any meaningful results for the terrarium.
Using some lamps
which are often used in reptile keeping as examples, I want to show
that CCT and CRI can be misleading as a measure for sun-like light.
If one compares the spectra of four lamps with a CRI over 90, it becomes
clear that a high CRI does not guarantee a solar-like spectrum (Figure
10).
- Only the Iwasaki
EYE Color Arc metal halide lamp from Eye Lighting International
(6,500K, CRI 96) shows a stunningly solar-like spectrum – including
the UVA region, between 350 nm and 400 nm.
- The Z-Power LED,
model “Natural White”, from Seoul Semiconductors has a very
high CRI (CRI 93, 4,000 K). But the spectrum is restricted to
wavelengths between 430 nm and 700 nm. The range from 350 nm
to 430 nm, that is visible for reptiles, is not covered.
- An incandescent
lamp, as a thermal light source, has by definition a CRI of
nearly 100 – even though it is hardly possible to distinguish
a blue thread from a black one, owing to the paucity of blue
light. Its spectrum bears little resemblance to the solar spectrum.
- The Narva BIOvital
fluorescent tube (5,800 K, CRI > 90) is often recommended as
first-class reptile lamp, but emits only small amounts of UVA
visible to reptiles. Compared to sunlight, it shows a very discontinuous
spectrum that does not differ much from the spectrum of a normal
triphosphor lamp such as the Osram Lumilux 840. (See figure
7.)
|
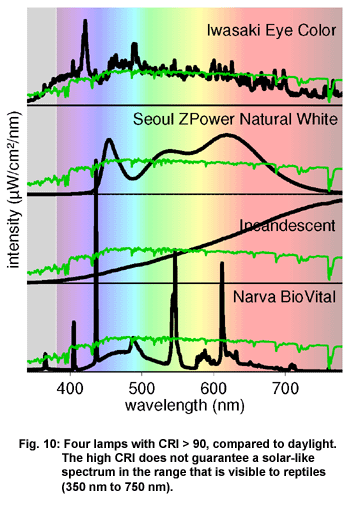 |
Nearly
all available lamps emit light that is white to the human eye.
Their colour coordinates in the colour triangle of man lie on the Planckian
locus with colour temperatures between 2,700 K and 6,500 K. Figure 11
shows the co-ordinates of a selection of the light sources already featured
in this article.
For reptiles this is not necessarily the same. A colour temperature
of between 4,000 K and 6,500 K printed on the package of a lamp does
not guarantee that the light will create a white, solar-like colour
impression for the reptile.
A Colour Space
for Reptile Vision
The concept of colour space
can be transferred to the tetrachromatic colour vision of reptiles (KELBER
et al. 2003).
In this case the relative
stimuli of four cones have to be considered, that is, a point drawn
in a x-y-z colour space. A colour pyramid replaces the colour triangle;
the extra dimension is due to the UV component of the light. The Planckian
locus is also in three-dimensional space since black body radiation
also has a UV component.
Lamps with an unbalanced spectrum will stimulate the four cones unevenly
and therefore deviate from the Planckian locus in the direction of the
spectral colours. Their light will seem coloured to the reptile - it
is no longer white.
Only the spectra of the halogen
lamp and daylight are nearly identical to the spectra of Planckian radiators.
Therefore their colour coordinates lie close to the Planckian locus
at 2,700 K and 5,100 K. All other light sources deviate more or less
from the Planckian locus and must appear coloured to a reptile.
Figure 12 (below) is a construction
of a colour pyramid for the hypothetical "archetypal reptile eye"
with 4 cones having the sensitivities illustrated above in Figure 6.
(This colour pyramid is therefore also only hypothetical; not all reptiles
are tetrachromats, and even amongst those which are, individual reptile
species have cones with different spectral sensitivities. But the principle
is valid.)
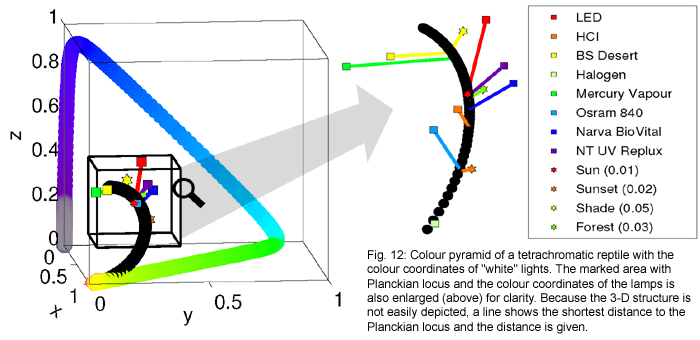
The following features are
of interest:
- Only man cannot distinguish
the light in the shade of a tree or in the forest from a Planckian
radiator. For reptiles these appear slightly coloured.
- The white LED has a very
restricted spectrum which stimulates the blue and green cones, but
not the UV or red cone. This is also the case for light with 500 nm
wavelength (turquoise). Therefore the colour coordinate of this LED
is dislocated far (distance 0.15) into the direction of turquoise,
to the reptile eye.
- The “Osram Lumilux 840”
does not stimulate the UV cone, because the lamp does not emit UV.
This is also the case for a Planckian radiator with low temperature.
Its colour coordinate consequently lies near 3,500 K but it is some
distance from the Planckian locus. What colour will it appear to a
reptile?
- The opposite happens with
the Lucky Reptile Bright Sun UV Desert metal halide lamp. Its high
proportion of UVA in the spectrum strongly stimulates the UV cone.
Its colour coordinate therefore lies at 9,000 K and is shifted into
the direction of violet/UVA.
- The same holds for the
mercury vapour lamp. The UVA mercury lines are very intense and strongly
stimulate the UV cone. Its colour coordinate lies near 8,000 K, but
dislocated into the direction of violet/UVA.
Whether the colour of terrarium
lamps has any influence on the husbandry success or the wellbeing of
reptiles has not yet been subject to any scientific study. But it is
undoubtedly clear that the manufacturer’s indication of CCT and CRI
only apply to human colour vision and therefore cannot indicate whether
the light’s colour seems like sunlight to reptiles.
There is no basis to the reasoning that one must limit the lamps to
specific CCT and CRI values, for the reptiles’ sake.
Reptile keepers should not imagine that specifications of 5,000 K and
CRI > 95 ensure a sun-like light source. Finding one is nevertheless
a desirable goal. Apart from colour vision, and UVB for Vitamin D production,
the effects of sunlight (especially its intensity) on the hormone system
and heat radiation for thermoregulation are important.
References
ALBERTS, A.C. (1989): Ultraviolet
visual sensitivity in desert iguanas: Implications for pheromone detection.
– Animal Behaviour 38(1): 129–137.
AMMERMÜLLER, J. & H. KOLB
(1996): Functional Architecture of the Turtle Retina. – Progress in
Retinal and Eye Research 15(2): 393–433.
ARNOLD K. E. & C. NEUMEYER
(1987): Wavelength discrimination in the turtle Pseudemys scripta elegans.
– Vision Research 27(9): 1501–1511.
BOWMAKER, J. K. & E. R. LOEW
(2005): The cone photoreceptors and visual pigments of chameleons. –
Journal of Comparative Physiology A 191(10): 925–932.
DECASTRO, F. (2000): Light
spectral composition in a tropical forest: Measurements and model. –
Tree Physiology 20(1): 49–56.
ENDLER, J. A. (1993): The
Color of Light in Forests and its Implications. – Ecological Monographs
63(1): 1– 27.
ELLINGSON J. M., L. J. FLEISHMAN
& E. R. LOEW (1995): Visual pigments and spectral sensitivity of the
diurnal gecko Gonatodes albogularis. – Journal of Comparative Physiology
A 177(5): 559–567.
FEDERER, C. A. (1966): Spectral
Distribution of Light in the Forest. – Ecology 47(4): 555–560.
FLEISHMAN, L. J., E. R. LOEW
& M. LEAL (1993). Ultraviolet vision in lizards. – Nature 365: 397.
HORN, H.-G. (2004): Beleuchtung
im Vivarium. – S. 168–224 in K. SAUER, B. STECK, H. SCHUCHART & H.-G.
HORN: PraxisRatgeber Vivarienbeleuchtung: Das richtige Licht in Aquarium
und Terrarium. – Chimaira, Frankfurt am Main.
KELBER A., M. VOROBYEV &
D. OSORIO (2003). Animal colour vision - behavioural tests and physiological
concepts. – Biological Reviews 78: 81–118.
KOBER I. & U GEISSEL (2006):
Grundlagenwissen Terrarienbeleuchtung: Ein Schlüssel zur erfolgreichen
Haltung. – Terraria 1: 6–16.
LEAL, M. & L. J. FLEISHMAN
(2002): Evidence for habitat partitioning based on adaptation to environmental
light in a pair of sympatric lizard species. – Proceedings of the Royal
Society of London – Biological Sciences 269(1489): 351–359.
LEBAS, N. R. & N. J. MARSHALL
(2000): The role of colour in signalling and male choice in the agamid
lizard Ctenophorus ornatus. – Proceedings of the Royal Society of London
– Biological Sciences, 267(1442): 445–452.
LOEW E. R., L. J. FLEISCHMAN,
R. G. FOSTER & I. PROVENCIO (2002): Visual pigments and oil droplets
in diurnal lizards: a comparative study of Caribbean anoles. – Journal
of Experimental Biology 205: 927–938.
PRZYREMBEL, C., B. KELLER
& CHRISTA NEUMEYER (1995): Trichromatic color vision in the salamander
(Salamandra salamandra). – Journal of Comparative Physiology A 176(4):
575–586.
RÖLL B. (2000). Carotenoid
and retinoid – two pigments in a gecko eye lens. – Comparative Biochemistry
and Physiology Part A: Molecular & Integrative Physiology 125(1): 105–112.
ROTH L. S. V. & A. KELBER
(2004): Nocturnal colour vision in geckos. – Proceedings of the Royal
Society of London - Biological Sciences 271(Suppl 6): S485–S487.
SCHANDA J. (2007): Colorimetry:
Understanding the CIE System. – Wiley, Hoboken, New Jersey.
SILLMAN A. J., V. I. GOVARDOVSKII,
P. RÖHLICH, J. A. SOUTHARD & E. R. LOEW (1997): The photoreceptors and
visual pigments of the garter snake (Thamnophis sirtalis): A microspectrophotometric,
scanning electron microscopic and immunocytochemical study. – Journal
of Comparative Physiology A 181(2): 89–101.
TAKENAKA N. & S. YOKOYAMA
(2007): Mechanisms of spectral tuning in the RH2 pigments of Tokay gecko
and American chameleon. – Gene 399(1): 26–32.
|